By Catherine Jomary, Ph.D. and Luis Martinez Darriba, MSc
Introduction
Since the emergence of COVID-19 mRNA vaccines, significant manufacturing advances have been made for expanding applications of mRNA vaccines and therapeutics. Improvements in mRNA synthesis and purification, mRNA sequence modification and optimization, and the refinement of lipid nanoparticle (LNP) delivery systems have accelerated mRNA development for wide-ranging therapies – from cancer vaccines to gene therapy and infectious disease treatments. The small size of LNPs, their biocompatibility, and biodegradability make them an optimal vehicle to protect mRNAs and deliver them into cells.
Notably, correct mRNA encapsulation is essential for the potency and stability of therapeutic products and vaccines. However, efficient mRNA-LNP encapsulation processes are complex, and their specific risks need to be addressed when selecting a manufacturing platform suitable for GMP production. Key manufacturing factors for LNP encapsulation include the lipid composition and the mRNAs mixing formulation method.
Understanding lipids
LNPs appear as inverted micelles where most cationic/ionizable lipids occupy the inner core (aqueous core) and an outer hydrophobic lipid layer. The positively charged ionizable lipids (at low pH) interact with the anionic mRNA during particle formation (see Figure 1 and Table 1). The lipid layer can be composed of various lipids, including phospholipids, cholesterol, and sometimes other lipid components, such as PEGylated lipids (lipids modified with polyethylene glycol).
The choice of lipids and their ratios can influence LNP stability, delivery, efficiency, biocompatibility, and immunogenicity. They can also be engineered to have specific characteristics, such as size, surface charge, and targeting ligands, to improve their performance and enable targeted delivery of mRNAs to specific tissues or cell types (see Table 1). In addition, PEG-lipids are typically used to control the LNP particle size and can act as a steric barrier to prevent aggregation during storage. The different lipid molar ratios used during formulation determine LNP size, heterogeneity, and efficacy. Together with the mRNA, these LNP components can form particles of about 60–100 nm in size.
Figure 1: schematic representation of mRNAs-LNP structure.
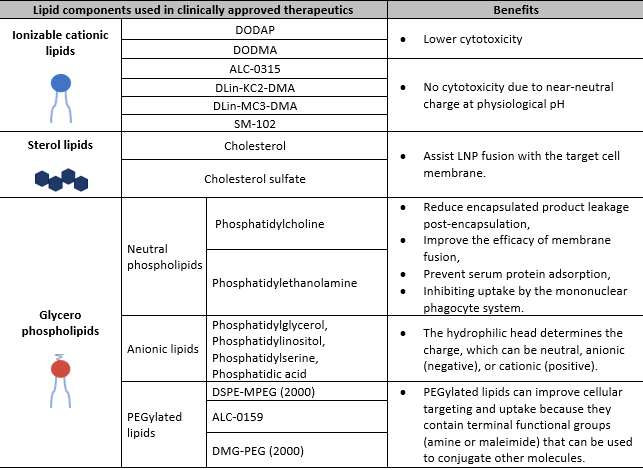
Table 1. Examples of lipid components used in clinically approved therapeutics.
Formulation of mRNA using LNP encapsulation can be performed using several manufacturing methods (Table 2). A manual method of transferring the lipids ethanolic mixture into the aqueous mRNA solution and injecting the ethanol mixture into the mRNA solution under constant stirring can be done in a laboratory setting. These labor-intensive processes result in heterogeneous LNP size distribution with low mRNA encapsulation efficiency. Their lack of scalability and reproducibility is unsuitable for GMP large-scale production.
Table 2. Comparison of mRNAs-LNP production methods
Scalability and robustness of mRNAs-LNPs encapsulation can be achieved by using continuous flow microfluidics devices. In those devices, at least two liquid streams are combined in a mixing chamber by either non-turbulent mixing or collision at high velocity. Control of the inlet streams - lipids in water-soluble solvent (organic phase) and mRNAs in aqueous solution (aqueous phase)- enable reproducible mixing conditions. The quality of mRNA- LNP encapsulation is mainly affected by:
- mixing chamber geometry
- fluid velocities
- streams flow stability
- the lipids composition.
The main mRNA encapsulation mixing chambers are T-junction, staggered herringbone (SHM), toroidal mixer, and confined Impinging jet mixer (see schematic examples in Figure 2). The geometry of the mixing chamber increases the interface between the two fluids.
Figure 2. Schematic examples of Microfluidic mixers for LNPs mRNA formulation.
A. In line T junction mixer B. Toroidal mixers C. Staggered herringbone micromixer D. Confined Impinging jet mixer.
The single-use–stainless steel equipment debate
To accelerate timelines from R&D bench scale processes to clinical and commercial GMP manufacturing, the selected mRNA-LNP encapsulation platform should facilitate process upscaling without risking process re-development.
Currently, two leading platforms are commercially available for GMP manufacturing mRNA encapsulation: the NanoAssemblr series, a single-use platform from Precision Nanosystems that uses toroidal mixing (a non-turbulent mixing), and the NanoScaler and NanoProducer series from Knauer, which are stainless-steel platforms (see Figure 3) that use impingement jet mixing (collision at high-velocity mixer). Both approaches can support efficient scalability.
Figure 3: Schematic of representative mixing flows path for both impingement jets mixer and toroidal mixer platforms
In the case of a toroidal mixer, the same microfluidic chamber geometry is used across the NanoAssemblr platform series. Hence, mRNA formulation is scalable across the platform from 1 mL/min to 800 mL/min flow. The GMP manufacturing platform is a single-use, closed process comprising benchtop equipment and a portable commercial skid. Several equipment units can also be used in parallel if a large production scale is required. As a single-use platform, the system does not require cleaning qualification or verification, and the risk of product contamination is minimized. However, warehouse space is needed to accommodate the demand for single-use consumables and associated logistics. This automatic continuous microfluidic platform shortens downtime during product changeover, increasing the manufacturing facility’s flexibility.
With impingement jet mixer technology, five different mixing chamber geometries are available across the NanoScaler and the NanoProducer series, which makes mRNA formulation scalable from R&D to GMP production scale (up to 2L/min flow with standard equipment). The GMP manufacturing platform is a stainless steel skid containing several HPLC pumping units and mixing chambers, converging into a standard outlet. It has the capacity for large-scale production.
*ATEX: The ATEX Directive 2014/34/EU European Directives covers equipment and protective systems intended for use in potentially explosive atmospheres.
Table 3. Comparison of mRNA- LNP formulation platforms advantages and disadvantages.
The choice of single-use versus stainless steel will impact the facility footprint (see overview of pros and cons in Table 3). Higher warehouse space will be required to accommodate single-use consumables and CIP and SIP systems are needed for stainless steel. In addition, the facility design and footprint are also impacted by the large-scale water production system required and the high level of liquid waste to be treated. Equipment choice will also depend on the mRNA manufacture scale and target, vaccines versus therapeutics, and the product clinical phase and commercial application. Manufacturing various mRNA products requires a multiproduct manufacturing facility with a flexible design.
Don’t forget regulations
Looking to the future, a key challenge for mRNA-LNP formulation resides in current regulatory ambiguities. Although the present regulatory framework does not strictly apply or mention mRNA therapeutics, the FDA and EMA have produced guidelines on the formulation of mRNAs with lipid nanoparticles. Preventive and therapeutic mRNA vaccines against infectious diseases are currently not considered gene therapy medicinal products, but they follow vaccine regulations as biological products. However, mRNAs produced from a recombinant source – linearized plasmid – that have a mechanism of action related directly to the product genetic expression of the mRNA sequence are considered a gene therapy product (EMA Article 2 of Regulation (EC) 1394/2007).
Lipid-based formulations of mRNAs, such as lipid nanoparticles, are essential to effectively and safely deliver the final drug products. Presently, defining whether LNPs are active ingredients or excipients is a challenge. For example, EMA has consistently defined LNPs in current commercial mRNA vaccine products as excipients. In contrast, they have been defined as excipients or as part of the drug substance depending on the products approved by the FDA. Harmonization between regulatory agencies and further clarification on LNP classification, terminology, and regulatory criteria is required to mitigate potential regulatory risks.
Manufacturers don’t have control over regulatory decisions, but they do have control over the processes they use. Efficient mRNA formulation requires selecting a microfluidic platform suited for the target mRNA LNP products scale, vaccines versus therapeutics, and the product’s clinical and commercial applications. Multiproduct facility design must accommodate the chosen microfluidic platform, and the associated utility footprint must permit cost-effective manufacturing of these novel mRNA therapeutic and vaccine products.
Published in Medicine Maker.